The MUonE Project (MUon ON Electron elastic scattering)
The MUonE experiment aims at a completely independent and very precise measurement of the leading hadronic contribution to the muon magnetic moment, achievable with a novel method.
Background. In classical electrodynamics an orbitating point-like electrically charged particle, of charge e, mass m and orbital angular momentum L, carries a magnetic dipole moment μL = (e/2m) L. This means that it behaves like a tiny magnet. However, even point-like particles at rest may have a magnetic moment μ that is aligned with their spin angular momentum S: μS = g (e/2m) S, where g is the so-called g-factor or dimensionless gyromagnetic ratio. Paul Dirac, using the theory of relativistic quantum mechanics, in 1928 predicted that the g-factor of elementary spin-1/2 particles, such as the electron or muon, is exactly g = 2 (to be compared with g = 1 in the case of the orbital angular momentum). At first, this seemed to be in agreement with atomic physics experiments.
In 1947 small deviations from the expectations were seen in the hyperfine structure of hydrogen and deuterium. A small deviation of the electron's g-factor from 2 was proposed as an explanation. In 1948 Julian Schwinger, using quantum electrodynamics (QED), calculated a tiny deviation of g from 2. His famous result, the so-called electron anomalous magnetic moment, lead to ae = (g − 2)/2 = α/2π, where α ≈ 1/137 is the fine structure constant. The further development of QED is very closely connected with ever more accurate predictions for g − 2, using perturbation theory. Today, the state of the art is the inclusion of corrections up to five so-called loops, i.e. virtual exchanges with photons and fermions (Schwinger did one loop, where just one photon is exchanged). In 2012, after many years' work (with the essential contribution of E. Remiddi and S. Laporta, from Alma Mater Studiorum – Università di Bologna), T. Kinoshita and collaborators finished the calculation of 12,672 Feynman diagrams; their five-loop contribution is proportional to (α/π)5, which is very small but still relevant when comparing experiment and theory. The calculated anomalous magnetic moment of the electron is, at present, ae(th) = 1.159 652 180 252 (95) × 10−3, while the experimental value is ae(exp) = 1.159 652 180 73 (28) × 10−3. The agreement is astonishing: accuracy is around 1 part per billion (109).
Much worse is the agreement between theory and experiment in the case of the muon, the heavier sibling of the electron (about 200 times heavier). The reason is that the muon's anomalous magnetic moment, aμ, is not only an effect from QED, but also contains significant contributions from the weak and strong forces of the standard model (SM) of particle physics. Moreover, any unknown particle or force in nature could contribute in addition to the known ones. The muon magnetic moment is one of the most precisely measured quantities (better than one part per billion). It is also calculable with extremely high precision in the Standard Model, hence it constitutes one of the most stringent tests of the theory. At present, the prediction of the Standard Model is aμ(th) = 1.165 918 10 (43) × 10−3, while the experimental measure leads to aμ(exp) = 1.165 920 89 (63) × 10−3, with a discrepancy of about 3.7 standard deviations. This is perhaps the only clear deviation from the Standard Model in the high energy physics landscape. There are hypotheses trying to explain this deviation as a quantum effect of new exotic particles. However it is still possible that it results from a systematic error either in the measurement or in the theoretical calculation.
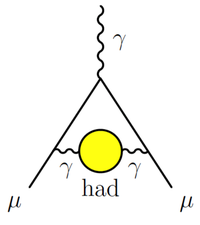
To progress on the experimental side, a new measurement is ongoing at Fermilab, targeting a reduction of the error by a factor of 4. A similar reduction of the theoretical error is desirable, but not simple at all. The largest error source is related to the evaluation of the hadronic contribution to the vacuum polarization, which is not calculable in perturbation theory. The current approach employs a large number of measurements from several different experiments to extract this contribution by a sophisticated combined fit. The alternative non-perturbative calculation of lattice QCD does not yet achieve the necessary precision.
The role of the MUonE Project. In this scenario the MUonE project was conceived, and Bologna played a key role in the original proposal. The idea was first presented at the workshop “Physics Beyond Colliders”, held at CERN in late 2016, and published in the European Physical Journal C soon after.
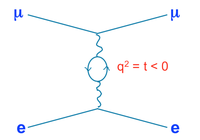
The MUonE proposal consists in evaluating the hadronic contribution to the vacuum polarization by measuring, with an unprecedented precision, the differential cross section of μ-e elastic scattering as a function of the transferred 4-momentum, using the intense muon beam available at CERN, with energy of 150 GeV, off atomic electrons of a light target.
The proposed apparatus is a sequence of detection stations, each one made of a passive element serving as target and active planes made of silicon microstrip detectors for tracking, with length of 1 metre and transverse dimensions of about 10 cm. The layout foresees an array of 40 such stations, followed by an electromagnetic calorimeter and a muon detector at the end.

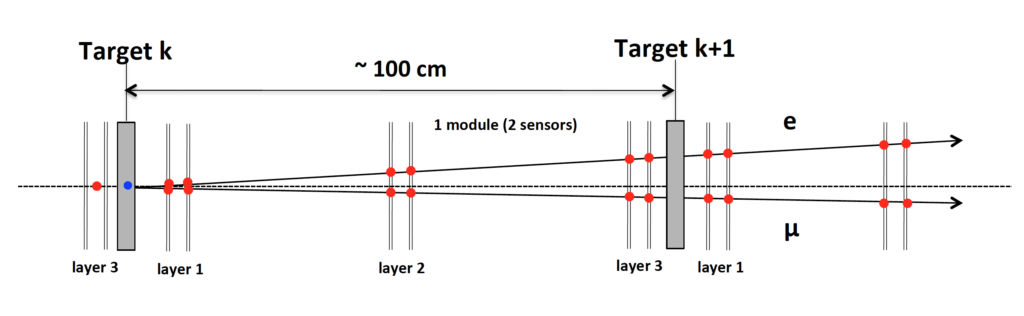
The MUonE project was submitted for approval to the CERN SPS Committee with the Letter-of-Intent presented in June 2019. CERN has approved a Test Run to be done at the end of 2021, which will be carried out with a couple of tracking stations and a prototype for the calorimeter.
Currently the Italian component is the largest in MUonE (Bologna, Pisa, Milano Bicocca, Padova, Perugia, and Trieste), but, as of today, English, Polish, American, Greek, and Russian colleagues are active members, besides CERN colleagues. In addition the involved Italian theorists are genuinely part of the enterprise (in particular our colleagues from Padova and Pavia), besides theorists from european institutions.
More details about the experiment can be found at the INFN site.
Gallery
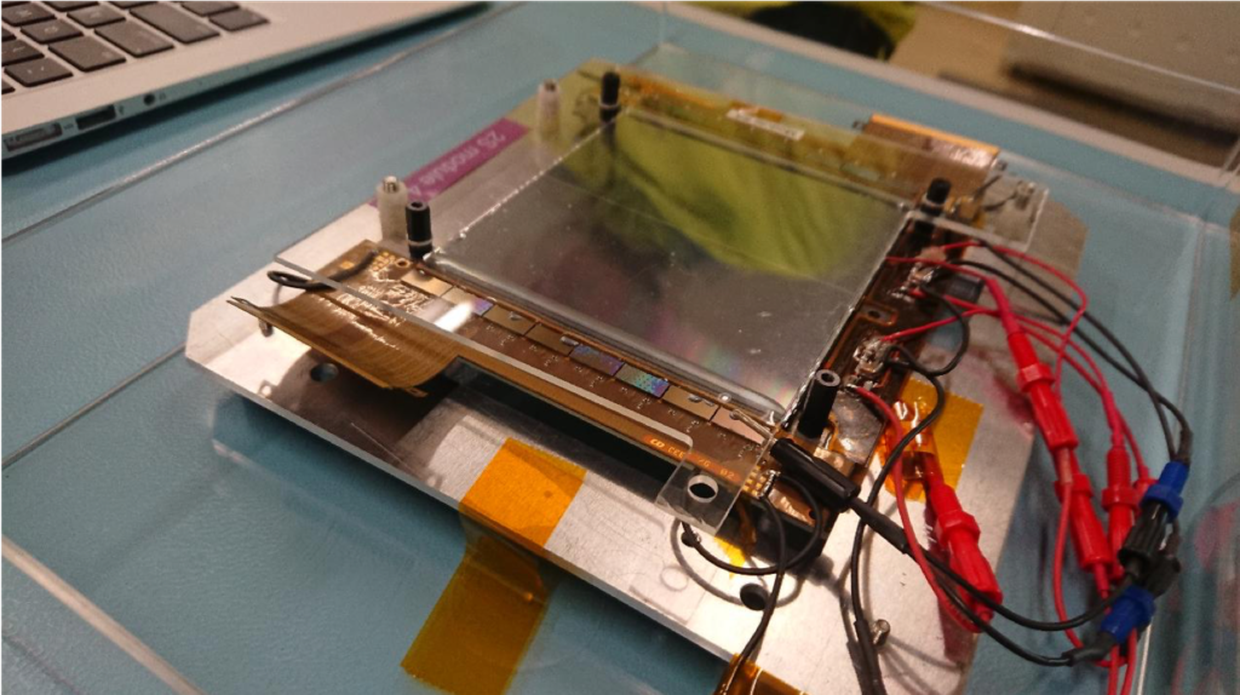
Tracker module
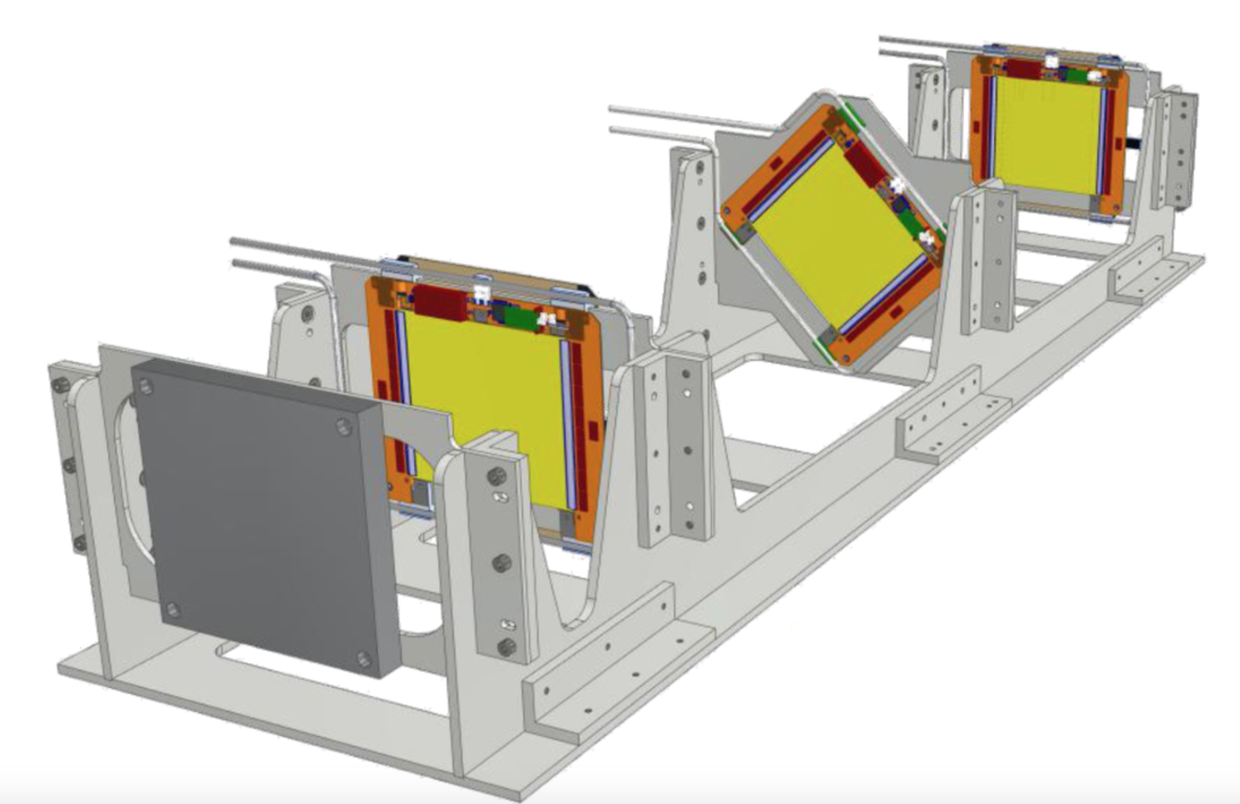
CAD layout of a station
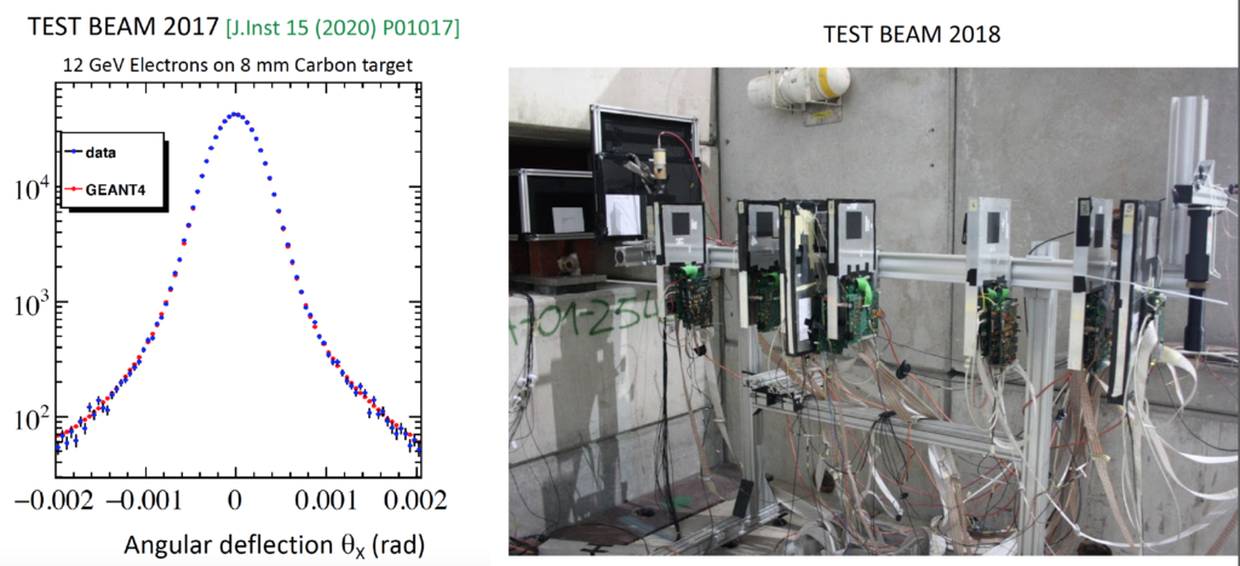
Test Beam 2018
DIFA members
Domenico Galli
Associate Professor
Claudia Patrignani
Associate Professor
Other Collaborators
-
Umberto Marconi
First INFN researcher
-
Giovanni Abbiendi
INFN researcher